By Clarissa Towle
Borders can be rough. Where two dissimilar materials meet, it may take some strain for them to exist in perfect harmony.
In a recent study in Nature, Molecular Foundry users Myoung Hwan Oh and Min Gee Cho described a way to grow extremely uniform nanoparticles whose atoms align perfectly with one another, and Foundry scientist Colin Ophus analyzed atomic-resolution electron micrographs to prove it.
The quality of the boundary between two different materials in a system has a huge impact on the system’s properties, such as electrical resistance. For example, the contacts between the tiny transistors and the connecting wires in a modern CPU computer chip need to be seamless, as a poor electrical connection between billions of transistors would lead to substantial energy loss — and overheated computers.
While these boundaries can now be carefully controlled in computer chips, it’s often challenging to exert this degree of control in other nanoscale materials, such as core-shell nanoparticles. Core-shell nanoparticles can be tens or hundreds of nanometers in size, and are composed of one type of material on the inside — the core — with another material forming a coating — the shell. This type of nanoparticle is often grown in a chemical bath, producing particles with a range of sizes and shapes, and in random relative orientations. In turn, this leads to a variety of boundary structures, known as “grain boundaries,” forming rough and randomized borders between the two crystal species in each nanoparticle.
In this study, researchers used several key design principles to achieve identical nanoparticles with harmonious borders, such as keeping the particles extra small and modifying their surface energy by changing the chemical environment during growth. These tricks allowed the team to successfully form Co3O4 nanocubes with Mn3O4 shells, with precisely-controlled grain boundaries and perfectly-aligned lattices, despite the two materials having very different preferred atomic spacings.
The design principles for this study lay the groundwork to achieve this control in other nanoscale systems, and may lead to the development of new nanocrystalline materials for use in chemical catalysis or electronics. The work has already evolved into multiple ongoing user projects at the Foundry.
At Berkeley Lab, this work was supported by the Physical Chemistry of Inorganic Nanostructures Program within the Materials Science Division and the National Center for Electron Microscopy (NCEM) at the Molecular Foundry. The work was performed in collaboration with Seoul National University, Hanyang University, Pohang University, the Korea Basic Science Institute, the University of California at Berkeley, and Stanford University.
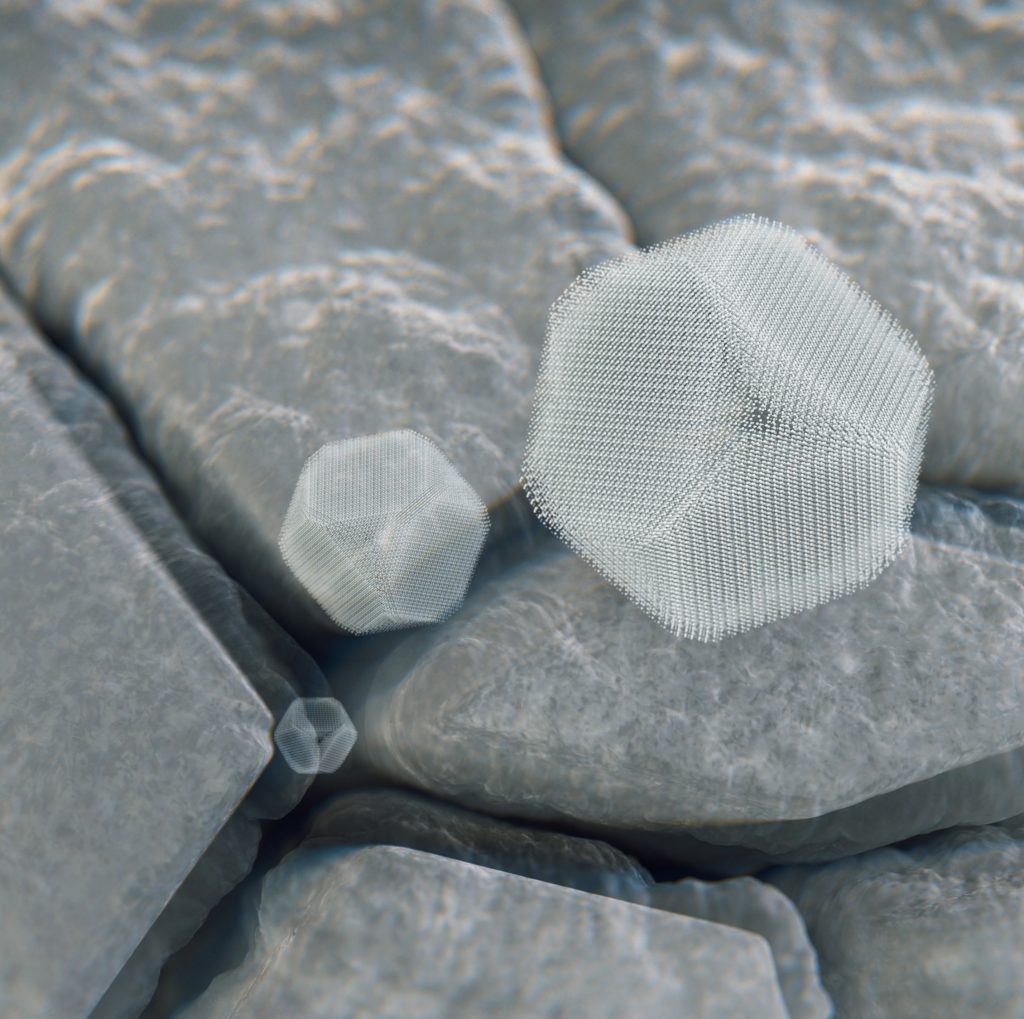