By Brooke Kuei
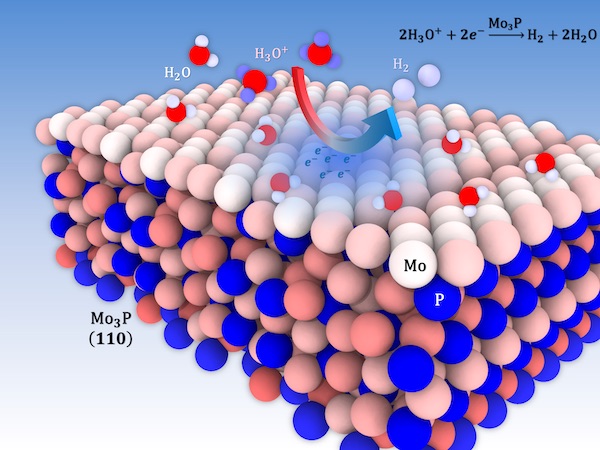
Global energy demand is expected to grow by nearly 30% from 2017 to 2040, with fossil fuels still making up the majority of energy supplies. Hydrogen fuel has emerged as an alternative energy source for sustainably meeting the world’s energy demands due to its high energy density and zero carbon emissions.
One “clean” way to make hydrogen fuel is to electrochemically split water into hydrogen and oxygen. Breaking down water requires an efficient catalyst, a material that increases the rate of a chemical reaction, but current catalysts such as platinum are scarce and expensive. In a study published in Advanced Energy Materials, Molecular Foundry scientists and their collaborators from the Illinois Institute of Technology found that trimolybdenum phosphide is a low cost, abundant alternative for hydrogen production.
“Our collaborators at Illinois Institute of Technology discovered a high efficiency catalyst, and we used theory to explain why it performs so well,” said Artem Baskin, project scientist at Berkeley Lab’s Molecular Foundry. “Using density functional theory (DFT), we simulate the material as a thick slab and investigate its properties throughout the slab and at the surface.” Their collaborators at Illinois Institute of Technology include assistant professor of chemical engineering Mohammad Asadi and his graduate student Alireza Kondori.
The researchers at Illinois Institute of Technology found that trimolybdenum phosphide requires only a small amount of energy in order for the reaction to occur. Molecular Foundry theorists explained this by calculating the material’s work function, the amount of energy needed to remove an electron from the surface of the material.
“Imagine that you have a reaction energy that sits at some level, like a rung on a ladder, and then you have the work function of the material – where all its electrons are – on a rung below it,” explained David Prendergast, Facility Director of the Theory of Nanostructured Materials at the Molecular Foundry. “The smaller the gap between the two rungs, the better.” For trimolybdenum phosphide, this gap is nearly as small as it is for platinum, the current standard for water splitting catalysts.
Another quality of trimolybdenum phosphide that helped explain its good performance is that it has a high density of molybdenum active sites on its surface. “By looking at how charge is distributed in the material, we found that the surface molybdenum atoms are significantly reduced, meaning they are rich in electrons and are the ones contributing to charge transfer,” said Baskin. Because the surface of a catalyst material is where reactions will occur, the high density of reduced molybdenum atoms on the surface of this material is likely responsible for its high catalytic activity.
Asadi and his team also discovered that this material’s ability to have hydrogen “stick” to it is just right. “If the hydrogen sticks to the material too easily, a lot of hydrogen may stick to the surface but then will never leave, and the catalyst only works once. If the hydrogen doesn’t stick enough, then the reaction won’t happen,” explained Baskin. Trimolybdenum phosphide interacts with hydrogen in such a way that sufficient hydrogen atoms can stick to the surface and react, but are able to leave and allow room for new reactants.
The Illinois researchers also found that the material is very stable (after running it for forty hours, the catalyst did not degrade) and it is conductive (so the electrons have a way of moving to the external circuit).
Baskin emphasized that although theory is often used to predict materials, in this case it played the important role of explaining the origin of high performance in a new, experimentally discovered catalyst.
Now that the high performance of trimolybdenum phosphide has been discovered, the team plans to study the same material for its application to carbon dioxide reduction.
The calculations for this study used the supercomputing resources at the National Energy Research Scientific Computing Center (NERSC).